Faculty
Listing
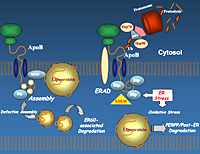 |
Khosrow
Adeli
Our laboratory
is studying the links between ER stress and the folding, ER translocation,
and intracellular degradation of apolipoprotein B (apoB), a very
large 4536 amino acid protein expressed in hepatocytes. ApoB is
a very important protein in regulating human cholesterol and fat
metabolism, and its hepatic production is increased in patients
with diabetes and cardiovascular disease (thus the interest in studying
mechanisms regulating its biosynthesis). ApoB is a highly hydrophobic
protein that requires co-translational lipidation for correct folding
of the emerging nascent chain in the ER. Impaired ER translocation
or folding of nascent apoB (e.g. in the absence of sufficient lipid
supply) leads to accumulation of the protein in the ER and rapid
degradation. We plan to modulate the lipidation and folding state
of nascent apoB chains and determine the upstream effects on ER
translocation and apoB mRNA translational efficiency. Based on recent
preliminary data (suggesting that misfolded apoB itself induces
ER stress), we also plan to determine the potential role of misfolded
nascent apoB in inducing ER stress via PERK signaling. Accumulation
of folding-incompetent proteins in the ER can normally disrupt ER
and activate the unfolded protein response (UPR). UPR activates
an ER resident transmembrane protein: double-stranded RNA-activated
protein kinase (PKR)-like ER kinase (PERK), which phophorylates
eIF2alpha. Collectively, these studies will help us delineate the
links between apoB misfolding, PERK signaling and upstream translational
control of apoB mRNA.
Website: http://www.sickkids.ca/Research/Adeli-Lab/index.html
Departmental Affiliation(s): Biochemistry
|
|
 |
Avi
Chakrabartty
In Alzheimer's
disease, insoluble fibrils composed of Ab peptides accumulate to
form senile plaques. A long-standing hypothesis has been that these
fibrils are neurotoxic and cause Alzheimer's disease. Recently,
however, a non-fibrillar soluble oligomer of Ab has been found to
inhibit long-term potentiation, a process that is critical for learning
and memory. These findings implicate soluble Ab oligomers in the
pathogenesis of Alzheimer's disease.
We have found
that soluble Ab oligomers can be formed and stabilized for extended
periods of time. Through kinetic experiments we are uncovering how
the oligomers form and then further assemble into more complicated
structures that culminate in amyloid fibril formation. Current projects
include the following: (a) to use sedimentation methods to determine
size of Ab oligomers, (b) characterize the structure of Ab oligomers
using biophysical techniques, (c) determine whether Ab oligomers
form under physiological conditions, (d) determine whether Ab oligomers
are toxic to neurons and neuron-like cells.
Website: http://www.oci.utoronto.ca/institutes/html/oci/msb/chakrabartty.html
Departmental Affiliation(s): Medical Biophysics, Biochemistry
|
|
 |
Tigran
Chalikian
Understanding
molecular forces, including hydration, that stabilize various protein
conformations is vital for our ability to develop strategies for
tackling the protein folding problem. One approach is to combine
thermodynamic and structural properties of proteins in an attempt
to identify and thermodynamically characterize a variety of interactions
that stabilize/destabilize a given protein state. In line with this
strategy, we use high precision densimetric, ultrasonic velocimetric,
calorimetric, and spectroscopic techniques to characterize the initinsic
and hydration properties of a startegically selected set of globular
proteins in their native and denatured states. In these studies,
which represent the initial step of our research program, we investigate
structurally well characterized, relatively small globular proteins.
As the work progresses, we gradually expand our experimental targets
to additional proteins to fully exploit the powers that the combination
of volumetric, calorimetric, and spectroscopic techniques provides
for understanding the role of individual molecular interactions
in protein folding.
Website: http://www.pharmacy.utoronto.ca/graduate/faculty/chalik.jsp
Departmental Affiliation(s): Pharmacy
|
|
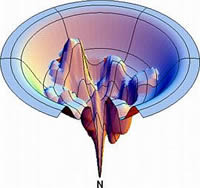 |
Hue
Sun Chan
A hierarchy
of heteropolymer models of various complexities are being developed
to capture the essential physics of protein folding. These constructs
allow for a broad coverage of conformational space -- in some cases
also sequence space -- at a level of relative mathematical rigor
currently not achievable in higher-resolution protein models. Physical
insights are being gained into general features of protein folding,
including calorimetric two-state cooperativity, chevron plots, and
the effects of temperature and denaturant dependencies of hydrophobic
interactions on native stability and folding/unfolding kinetics.
The polymer-physics-based sequence-structure mappings afforded by
some of these models are also applied to theories of evolutionary
landscapes. A closely related research area is statistical mechanical
theories and atomic simulations of aqueous solvation. This effort
aims to attain a fundamental understanding of hydrophobic and other
solvent-mediated interactions by simulating potentials of mean force
of model-compound solutes in explicit water.
Website: http://biochemistry.utoronto.ca/chan/bch.html
Departmental Affiliation(s): Biochemistry (primary), Molecular Genetics
|
|
 |
Alan
Davidson
My laboratory
is investigating the protein folding problem using both in vitro
experimental and bioinformatic approaches. We are using the SH3
domain as a model system to address a variety of issues in the field
of protein folding. SH3 domains are 60 residue protein-protein interaction
modules, which are found in a wide variety of larger proteins involved
in myriad intracellular signaling pathways. Our approach to studying
protein folding is to perturb atomic interactions within the protein
using mutagenesis, and then to characterize the changes in thermodynamic
stability, protein folding kinetics, and peptide binding function
caused by these perturbations using a variety of biophysical techniques.
By the analysis of large numbers of mutants, we are able to illuminate
the mechanisms by which proteins fold. We also use rigorous statistical
analyses of protein sequence alignments to aid in interpreting our
experimental results, and in predicting the effects of amino acid
substitutions.
Website: http://lambda.med.utoronto.ca/Davidson/
Departmental Affiliation(s): Biochemistry, Molecular Genetics
|
|
 |
Charles
Deber
Genetic mutations
in the transmembrane (TM) helices of proteins underlie the causes
of many human diseases, including several forms of cancer, diabetes,
cystic fibrosis, and muscular dystrophy. These domains have been
characterized in our lab by several criteria, including composition,
sequence, and hydrophobicity. Using this background knowedge, we
are now focusing on protein folding as follows: (i) Bioinformatics
approaches to disease-causing mutations in membrane proteins - As
polar TM mutations produce a striking variety of genetic diseases,
we are determining the 'phenotypic propensities' and scope of mutation
patterns of both polar and non-polar residues in TM domains, in
conjunction with how inter-helical polar side chain-polar side chain
hydrogen bond formation/loss impacts on protein folding within membranes.
(ii) Hydrophobic patches in soluble proteins as nucleation sites
for folding - Evidence from the hydrophobicity algorithm TM Finder
suggests that short hydrophobic sequences in soluble proteins are
membrane-insertion competent yet too short to act as TM segments.
We are investigating proteins with two or more such patches to determine
their role in guiding tertiary structure development.
Website: http://www.sickkids.ca/aboutsickkids/directory/people/d/charles-deber.html
Departmental Affiliation(s): Biochemistry
|
|
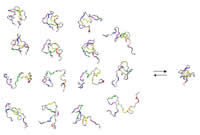 |
Julie
Forman-Kay
For a full
understanding of protein folding and stability, characterization
of unfolded and other disordered states of proteins is required.
In addition, studies of disordered states are critical in order
to probe the range of biologically relevant conformations and their
roles in protein recognition since chaperones, transport proteins
and proteolysis machinery recognize disordered states and many proteins
are unstructured under native conditions, particularly in the absense
of binding targets. Our work is focussed on the characterization
of disordered states of protein using nuclear magnetic resonance
(NMR), other spectroscopic and computational approaches. Our goals
are to characterize preferential structure in disordered states,
measure kinetic and thermodynamic properties, calculate conformational
ensembles of disordered states and perform computational simulations.
In addition, we are investigating the structures and dynamics of
protein chaperones and their interactions with disordered proteins.
Website: http://www.sickkids.ca/aboutsickkids/directory/people/f/julie-forman-kay-staff-profile.html
Departmental Affiliation(s): Biochemistry
|
|
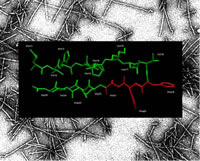 |
Paul
Fraser
Alzheimer Amyloid
- Structure and Function
Research is being conducted to determine the physical organization
and the mechanisms by which the amyloid-b (Ab) protein is assembled
into amyloid fibrils. This data is being correlated with functional
studies to ascertain, for example, to neurotoxicity of the Ab fibrils
to provide a comprehensive analysis of the contribution of amyloid
plaques to Alzheimer pathogenesis. Overall these investigations
are directed towards the develop of new therapeutic strategies to
control amyloid deposition.
Presenilins
- Biochemistry and Structure
Research into this novel family of proteins is aimed at understanding
their biochemistry as it relates to the key pathological features
of Alzheimer's disease, plaques and tangles. This involves the examination
of amyloid processing as well as the roles of functional ligands.
In addition, structural studies of key domains are being pursued
to provide a complete analysis of presenilin structure and function.
Website: http://medbio.utoronto.ca/faculty/fraser.html
Departmental Affiliation(s): Medical Biophysics
|
|
|
John
Glover
In the
Glover lab, yeast is used as a model system to examine the roles
of molecular chaperones in protein folding in different cellular
compartments. One focus of the lab is the oligomeric ATPase Hsp104.
The unique ability of Hsp104 remodel aggregated proteins is thought
to be the basis of its vital contribution to thermotolerance in
yeast and to the stability of various yeast prions- novel protein-based
genetic elements. The current research on Hsp104 involves establishing
the key features of Hsp104-mediated protein extraction from aggregates
and its interactions with yeast prion proteins. The Glover lab is
also studying the chaperones in the yeast endoplasmic reticulum.
Perturbation of protein folding homeostasis in the ER elicits a
specific stress response. Chronic ER stress is thought to lead to
cardiovascular complications in some types diabetes and the genetic
disorder hyperhomocysteinemia in humans. The role of chaperones
in maintenance of ER function is being investigated.
Website: http://biochemistry.utoronto.ca/glover/lab/
Departmental Affiliation(s): Biochemistry
|
|
|
Claudiu Gradinaru
The Gradinaru biophysics laboratory employs advanced laser and detection technology to capture structural and functional dynamics of individual biomolecules. Single-molecule techniques are widely applied to the study of protein folding, enzymes, chemical receptors and biosensors, due to a unique capability to "see" beyond the intrinsic disorder and complexity of biological systems and to better connect with theory. The group has designed and built fluorescence microscopes capable of simultaneous recording of multiple properties of weak optical signals emitted by single probes. Various spectroscopy and imaging capabilities are available in the lab, such as multiparameter single-molecule fluorescence, burst, FRET, FRAP, FCS, PCH and multichannel WF/TIRF microscopy. Currently, we are focused on the study of rapidly fluctuating structures of intrinsically disordered protein and of the inhibitory peptidic drugs against the transcription protein Stat3. We are also investigating the structure of thermosensitive lipid-coated polymeric beads for drug delivery and the crowding-induced anomalous diffusion of proteins in the nucleus of live cells.
Website: http://www.utm.utoronto.ca/~w3gradin/
Departmental Affiliation(s): Physics, Institute for Optical Sciences
|
|
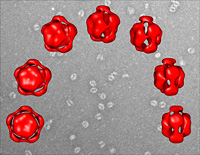 |
Walid
Houry
The ultimate
aim of our projects is to address the fundamental question of how
molecular chaperones modulate protein folding in the cell. To this
end, we study the role of molecular chaperones in maintaining protein
homeostasis using E. coli and yeast as model systems. We are also
mapping what we call the chaperone interaction networks in yeast
with the ultimate aim of identifying the rules that govern protein
folding processes in the cell. Our group employs a battery of approaches
including biochemical, biophysical, proteomics, and bioinformatics
tools. The two chaperone families that we are concentrated on include
the Hsp100 family and the Hsp90.
Website:
http://biochemistry.utoronto.ca/houry/Labpage
Departmental Affiliation(s): Biochemistry
|
|
 |
Mitsu
Ikura
My laboratory
is interested in elucidating structure, dynamics, and interaction
of biological macromolecules in order to understand, at the molecular
and structural level, how they function in the cell. Of particular
interest are proteins involved in calcium signaling, cell-cell interactions
and transcriptional regulation. Our recent studies highlighted a
significance of protein folding events in those cellular functions.
For example, our studies on the calcium signaling protein STIM1
(stromal interaction molecule 1) revealed that the activation of
STIM1-mediated store-operated calcium entry (SOCE) employs a molecular
mechanism in which protein unfolding coupled with oligomerization
process plays a crucial role. STIM1 and its target calcium channel
termed Orai1 are both implicated in genetic diseases such as severe-combined
immune disease (SCID). Our ultimate goal is to find ways to cure
this and related human diseases including cancer. Our primary tool
is NMR spectroscopy, but we also use a variety of biophysical and
molecular biology techniques, including X-ray crystallography and
in vivo fluorescence imaging microscopy.
Website: http://nmr.uhnres.utoronto.ca/ikura
Departmental Affiliation(s): Medical Biophysics
|
|
 |
Lewis
Kay
NMR methods
are developed and applied to study a wide range of biophysical problems.
In the area of protein folding and molecular dynamics new triple
resonance NMR experiments are designed to probe residual structure,
dynamics and thermodynamics of unfolded and partially unfolded states.
Relationships between dynamics and thermodynamics are being developed
and applied to unfolding events.
Website: http://abragam.med.utoronto.ca/UTNMR.html
Departmental Affiliation(s): Molecular Genetics, Biochemistry,
Chemistry
|
|
 |
Clifford
Lingwood
Our studies
related to protein folding fall into two categories.
Firstly, the
sulfoglycolipid (SGL) binding of the N-terminal ATPase containing
domain of hsp70. We have shown that SGL binding to this domain inhibits
hsp70 ATPase activity and are currently investigating the effect
of this binding on hsp70 chaperone function in several assays: a)
SV40
replication; b) in CFTR cell surface trafficking.
The second
area involves the intracellular trafficking of CFTR and how this
relates to
glycosphingolipid/cholesterol containing rafts. The studies also
intertwine with the potential regulation of hsp70 by SGLs since
there are themselves components of glycosphingolipid rafts and hsp70
is intimately involved in CFTR chaperoning and degradation. Our
studies are aimed at determining whether the cellular SGL content
has an influence on the ability of the misfolded mutant deltaF508CFTR
to traffic to the cell surface. We have synthesized a soluble SGL
mimic which inhibits hsp70 ATPase and are investigating its effect
on deltaF508CFTR folding and trafficking. We have also shown that
the 3'sulfogalactose moiety of SGLs is in part structurally analogous
to tyrosine phosphate and therefore are examining any possible role
for tyrosine phosphate motifs in either mimicking or regulating
these interactions.
Website: http://www.sickkids.ca/Research/Lingwood-lab/index.html
Departmental Affiliation(s): Laboratory Medicine & Pathobiology,
Biochemistry
|
|
|
Don
Mahuran
Extra- and
intra- cellular macromolecules, many of which contain carbohydrates,
are degraded in a stepwise manner in the lysosome and their components
subsequently recycled. Specific lysosomal exoglycosidases are involved
in this process. If one of these enzymes is deficient, partially
degraded macromolecules are trapped in the lysosomes, resulting
in disease. Together, lysosomal storage diseases (LSDs) occur at
a rate of ~1 in 5000 births, making them a significant health burden.
Like secretory proteins, lysosomal enzymes are synthesized in the
endoplasmic reticulum (ER). Once they fold and pass the ER’s
quality control system they are transported through the Golgi and
targeted to lysosomes. Many LSDs are caused by the inability of
the newly synthesized mutant protein to fold or remain folded in
the ER, leading to enzyme deficiencies and substrate storage. We
postulate that clinical symptoms are exacerbated by the presence
of the unfolded mutant protein itself, which initiates the unfolded
protein response (UPR) leading to ER-stress, and cell death. Currently
there are two major approaches to treat these disease, enzyme replacement
(ERT) and substrate reduction (SRT) therapy. The former costs ~$300,000/
yr./ patient, which limits availability, and the only compound presently
being used for the latter has unpleasant side effects that limit
its dosage/ efficacy. Neither of these approaches address the problems
caused by the UPR. Additionally, ERT is unable to deliver enzyme
to the CNS, or other organ compartments. We are developing enzyme
enhancement therapy (EET) as a new therapeutic approach. EET uses
small molecules that bind to and stabilize the properly folded form
of the enzyme, overcoming the destabilizing effects of the mutation.
EET-agents are known as chemical or pharmacological chaperones and
often are competitive inhibitors of the target enzyme. Additionally
we are investigating the pathways and mechanisms that mutations
in selected LSDs initiate to produce the UPR in cells.
Website: http://www.sickkids.ca/aboutsickkids/directory/people/m/don-mahuran-staff-profile.html
Departmental Affiliation(s): Laboratory Medicine & Pathobiology,
Medicine, and Institute of Medical Sciences
|
|
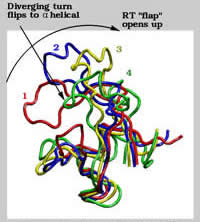 |
Régis
Pomès
We use structural
models derived from spectroscopic studies together with fundamental
physical laws governing atomic interactions to simulate the time
evolution of biomolecular systems. With modern supercomputers, these
molecular "cartoons" typically cover time scales of 10-15
to 10-8 s, a time span relevant to such important biophysical processes
as enzymatic reactions, molecular recognition and binding, ion transport
across biomembranes, and the proper folding and function of proteins.
The atomic resolution and the wealth of details provided by the
simulations make them a useful tool to complement experimental studies.
In collaboration with other research groups in Toronto and elsewhere,
we study equilibrium properties and dynamic fluctuations involved
in the function of soluble and membrane proteins, in folding-unfolding
processes, and in protein-ligand association. The attached figure
depicts collective motions in thermally-induced unfolding of the
N-terminal SH3 domain from drk.
Website:
http://www.pomeslab.com/
Departmental Affiliation(s): Biochemistry
|
|
 |
Gil
Privé
We are studying
the fundamental properties of protein-lipid and protein detergent
interactions with an emphasis on the thermodynamics of membrane
protein folding. We are particularly interested in characterizing
the detergent-solubilized state of membrane proteins. This is important
for the structural study of membrane proteins by crystallography
and NMR, but this also gives us insight into the intrinsic properties
of integral membrane proteins.
We have developed
a method to refold protein from the SDS-denatured state by the addition
of certain small amphipathic alcohols, such a 2-methyl-2,4-pentanediol.
We are currently trying to understand the physical basis for this
effect. We are looking the interactions of model peptides with a
series of detergents and cosolvents with calorimetric, spectroscopic
and molecular dynamic methods. We are also developing lipopeptide
detergents (LPDs), a fundamentally different class of amphiphile
designed to better mimic lipid bilayers. LPDs result in dramatic
increases in the thermostability of solubilized membrane proteins
relative to traditional detergents. We use de novo principles in
designing our LPD peptide sequences and structures.
Website:
http://xtal.uhnres.utoronto.ca/prive
Departmental Affiliation(s): Medical Biophysics, Biochemistry
|
|
 |
Scott
Prosser
We are interested
in protein folding mechanisms through solution state NMR studies,
of calmodulin, a key soluble protein involved in calcium mediated
signaling. By NMR, it is possible to track residue-specific chemical
shifts along the protein folding pathway and in so doing, reveal
potential folding intermediates, cooperative processes, and regions
where folding is initiated. We make use of additional biophysical
techniques to better understand the folding mechanism. For example,
by dissolving oxygen into the sample or by exploring solvent isotope
effects we can “see” solvent exposed surface area for
each residue, along the folding pathway. This helps us understand
the sequence of events associated with folding events. We also routinely
make use of pressure and biosynthetic labeling with 19F probes to
gain insight into the nature of the unfolded states, via NMR. This
approach can be extended to in vivo conditions, where viscosity
and crowding, plus binding partners often cause linewidths to increase
by a factor of 5 or more. Our solution is to “tag” target
domains in protein samples with robust and well-resolved monomethyl
groups such that NMR can be used to monitor folding events and post-translational
modifications, upon microinjection or trafficking of labeled proteins
into Xenopus oocytes or yeast cells.
Website:
http://fourier.utm.utoronto.ca
Departmental Affiliation(s): Chemistry, Biochemistry
|
|
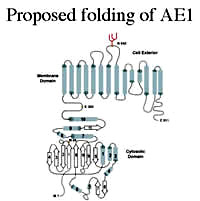 |
Reinhart
Reithmeier
My laboratory
is interested in the structure, function and assembly of membrane
proteins, with a particular focus on the anion exchanger (AE1) found
in erythrocytes and in acid-secreting intercalated cells in the
kidney. This membrane glycoprotein catalyses the electro-neutral
exchange of chloride and bicarbonate across plasma membranes and
is involved in bicarbonate transport, intracellular pH regulation
and acid secretion. Mutations in the AE1 gene cause a number of
human diseases, including Southeast Asian ovalocytosis (SAO) and
hereditary spherocytosis (HS) that affect red blood cells, and distal
renal tubular acidosis (dRTA) that affects acid secretion by the
kidney. In an extensive set of studies using transfected cells we
have shown that the mutations cause mis-folding of AE1 and impaired
trafficking to the cell surface. We are studying the interaction
of chaperones and other components of the quality control machinery
of the cell with normal and mutant forms of AE1 in order to determine
their role in the retention of AE1 mutants. High-throughput analyses
(membrane yeast 2-hybrid and proteomics) are being used to identify
the full complement of cellular proteins that interact with normal
and mutant forms of AE1. We are also carrying out biophysical and
NMR studies to determine the effect of mutations on the structure
and stability of the cytosolic domain of AE1 expressed in E. coli.
The overall goal is to determine the effect of mutations linked
to disease on the folding and trafficking of AE1 and the cellular
components involved in this process.
Website:
http://reithmeier-lab03.med.utoronto.ca/~reinhartreithmeier/lab/
Departmental Affiliation(s): Biochemistry
|
|
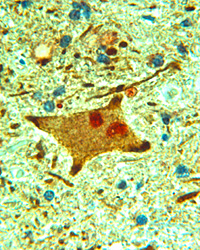 |
Janice
Robertson
Amyotrophic
lateral sclerosis, or Lou Gehrig’s disease, is characterized
by the presence of proteinaceous aggregates in motor neurons of
the brain and spinal cord, the neuronal type affected in the disease.
Our aim is to understand how these aggregates are formed and to
establish their relationship to the neurodegenerative mechanism.
The two major proteins of interest in our laboratory are the neuronal
intermediate filament protein, peripherin, and superoxide dismutase-1
(SOD1). Mutations within SOD1 account for ~3% of all ALS cases and
the mutant SOD1 protein has a propensity to misfold and to aggregate.
Peripherin aggregates are found in 80% of all ALS cases and transgenic
mice overexpressing peripherin develop a motor neuron disease. We
use molecular cell biology approaches, including primary cell culture,
transgenic mouse models of ALS and human pathological tissue, together
with protein-biochemical techniques to understand how a protein
aggregate leads to neuronal death.
Image:
Motor neuron in ALS spinal cord double-labeled with antibody to
peripherin (brown) and ubiquitin (red). Ubiquitin labels various
structures within diseased motor neurons, appearing as aggregated
material. Section is counterstained with haemotoxylin and eosin.
Normal control tissue is negative for peripherin and ubiquitin immunoreactivity.
X40 magnification.
Website:
http://icarus.med.utoronto.ca/patho/faculty.asp?FacultyID=425
Departmental Affiliation(s): Laboratory Medicine and Pathobiology
|
|
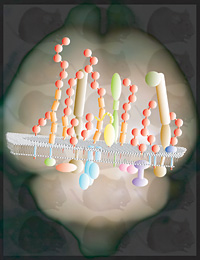 |
Gerold
Schmitt-Ulms
Our work contributes
to two strands of research at the interface of proteomics and neurodegenerative
disease research: the development of strategies for the study of
protein interactions and the application of these strategies to
address questions in the context of neurodegenerative disease research.
The laboratory is involved in collaborative activities with the
objectives to (i) understand the molecular assembly and activation
of
the presenilin complex in Alzheimer's disease; (ii) map the molecular
environment that hosts the conversion of the prion protein in
Creutzfeldt-Jacob disease; and (iii) elucidate molecular events
that underlie the intracellular deposition of Lewy bodies and cell
death in Parkinson's Disease and motor neuron degeneration in Amyotrophic
Lateral Sclerosis.
Image: Interactome map of the cellular prion protein following time-controlled
transcardiac perfusion crosslinking of mouse brains
(please see Nat. Biot. 22 (2004), 724-731, for details).
Website: http://individual.utoronto.ca/cpu/index.htm
Departmental Affiliation(s): Laboratory Medicine & Pathobiology
|
|
|
Simon
Sharpe
Our research
focuses on elucidating the structure and mechanisms of assembly
of macromolecular peptide/ protein complexes, with a particular
emphasis on membrane-active proteins involved in human disease.
Specific systems being investigated in the lab include: (1) Non-fibrillar
oligomers formed by amyloid peptides. These have been implicated
as the key cytotoxic agents in numerous neurodegenerative diseases,
and we are working to define the molecular mechanism of their activity
at cell membranes. (2) Early misfolding intermediates formed by
the mammalian prion protein (PrP). Conversion of PrP from a native
helical form to the misfolded, beta-sheet rich scrapie form is the
hallmark of prion diseases, such as Creutzfeldt-Jakob disease or
bovine spongiform encephalopathies. Understanding the structure
of oligomeric species formed early in the misfolding pathway will
shed light on the mechanisms of conversion and disease progression.
(3) Viral pore/channel proteins (viroporins). These proteins play
an important role in the life cycle of several viral pathogens,
including HIV-1, hepatitis C and dengue virus. We are studying the
structure and functional assembly of these proteins as a first step
towards development of new antiviral therapies.
Website: http://www.sickkids.ca/Research/Sharpe-lab/index.html
Departmental Affiliation(s): Biochemistry
|
|
|
Boris
Steipe
Website: http://biochemistry.utoronto.ca/steipe/
Affiliation(s): Biochemistry, Molecular Genetics
|
|
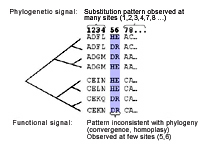 |
Elisabeth
Tillier
My lab is currently
working on the development of computational methods for the analysis
of protein sequences. Sequence information is exploding such that
a large sampling of the possible functional sequence space that
is explored through evolution is available for analysis. Analyses
of sequence alignments from protein families contain much information
on their function, structure and evolution. The development of accurate
analysis tools permits the prediction of amino acids involved in
protein folding, structure, function and even protein-protein interaction.
Current analyses rely on absolute conservation of amino acid sites,
where the methods we are developing also consider co-variation patterns
between amino acid sites within and between proteins. Analyses of
specific proteins of interest will allow us to infer the important
residues involved in protein structure and function and give clues
on the nature of disease involving these proteins and the potential
for designed targeting of particular residues by drugs. Additionally,
large scale high-throuput analyses of gene families may allow the
discovery of general biochemical principles of protein function.
We have also
been working in collaboration with Walid Houry (Biochemistry, University
of Toronto) in a study of the phylogenetic distribution of Molecular
Chaperones. The impetus of this work has been the conspicuous absence
of the GroEL chaperone in several sequenced genomes of Mycoplasma.
Using comparative genomic analyses we are investigating mechanisms
by which the bacteria can cope with the loss of such an important
protein folding chaperone.
Website: http://www.uhnres.utoronto.ca/tillier/
Departmental Affiliation(s): Dept. of Medical Biophysics
|
|
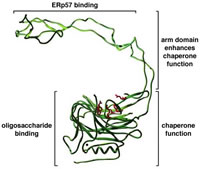 |
David
Williams
Protein folding
in vivo differs from the situation in a test tube in that nascent
polypeptides attempt to fold even before their entire length has
been synthesized. This should lead to aggregation. However, in vivo,
molecular chaperones recognize hydrophobic segments of non-native
polypeptides and, through regulated cycles of binding and release,
prevent aggregation and allow productive folding to occur. We study
protein folding within the the endoplasmic reticulum, a unique environment
wherein polypeptides are glycosylated, acquire disulfide bonds,
and undergo folding. Our emphasis is on the chaperones calnexin
and calreticulin; truly remarkable proteins that assist folding
by binding both to unfolded polypeptide segments and to oligosaccharide
chains. They also assist folding by recruiting a thiol oxidoreductase
to the unfolded substrate. To determine how these diverse functions
are organized and regulated, we use a combination of approaches
that include monitoring of folding in vivo, structure-function mutagenesis
studies, detection of novel folding factors, and reconstituting
folding in vitro with defined components.
Website: http://biochem17.med.utoronto.ca/%7Edavid_williams/lab
Departmental Affiliation(s): Biochemistry, Immunology
|
|
|
Shoshana
Wodak
My protein
folding related research includes the following topics:
1) Investigation of the amino acid sequence space available to protein
folds and in particular the ‘designability’ problem,
aimed at answering the questions of why certain protein folds (the
so-called super folds) have so many more sequences associated with
them than other folds. To tackle this issue we compute large families
of sequences that are compatible with sets of protein backbone folds
(retrieved from the PDB), using our CHARMM-based sequence design
procedure DESIGNER. Various parameters (including position specific
sequence variability, correlated sequence changes etc..) and properties
of the computed sequences are then analyzed in order to investigate
the constraints imposed on the sequence by the requirement to adopt
specific folds.
2) Folding and stability of proteins with restricted amino acid
alphabets. (e.g. IKEA). This is a collaboration with Donald Hilvert
(ETH, Zurich), who performs in-vivo selection of Chorismate Mutase
variants with restricted amino acid alphabets that have enzymatic
function. Using DESIGNER we perform the equivalent selections in-silico
and compare our results; we also use DESIGNER to guide the design
of DNA libraries that will produce a higher yield of functional/stable
proteins in vivo.
3) Development of new procedures for fold recognition and for the
prediction of protein-protein and protein-DNA interactions, using
structural information and families of amino acid sequences selected
by our sequence design procedure.
Website: http://biochemistry.utoronto.ca/wodak/bch.html
Departmental Affiliation(s): Biochemistry, Molecular Genetics
|
|
 |
Andrew
Woolley
Our research
is aimed at finding general strategies for the photo-control of
peptide and protein structure – and thereby activity. In doing
so, we gain fundamental knowledge about protein folding, and dynamics.
We also develop powerful tools for the analysis of complex biochemical
systems.
We have developed a series of chemical cross-linkers containing
azobenzene chromophores that undergo photochemical cis-trans isomerization.
Introduction of these compounds at judicially placed sites in peptides
an proteins can permit effective coupling between cis-trans isomerization
and conformational change (folding or unfolding) of the protein.
We have done extensive work on the photo-control of alpha-helical
systems since these are widespread and functionally important. Since
the photochemical isomerization event is very rapid (sub-picosecond),
helix formation can be tracked in detail over ps to ms timescales
using time-resolved spectroscopic techniques providing and unprecedented
level of information on the folding process.
We plan to extend this approach to studying and controlling conformational
changes in more complex systems. We are also trying to extend the
approach to permit in vivo introduction of such photo-switches.
Website: http://www.chem.utoronto.ca/staff/GAW/
Departmental Affiliation(s): Chemistry
|
|
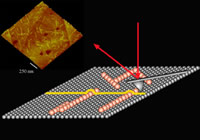 |
Christopher
Yip
Our group is
fundamentally interested in understanding how biomolecules self-assemble
hence our focus on single molecule biophysics. Self-assembly is
the formation of complex hierarchical structures from their individual
atomic or molecular elements without external intervention. By teasing
out key structural and chemical parameters, which often hinges on
detailed characterization of the interface between the constituent
molecules or aggregates, we can develop a set of "rules"
or precepts critical to efforts on molecular and protein engineering.
To study these mechanisms over all dimensional scales from individual
molecular complexes to the formation of two and three-dimensional
supramolecular assemblies, we are combining traditional approaches
such as circular dichroism, light scattering, and spectroscopy,
with high-resolution in situ scanning probe microscopy (SPM). Our
research programs also include computational approaches to simulating
protein assembly and a strong effort into the design, fabrication,
and application of functional single molecule imaging tools.
Website:
http://bigten.med.utoronto.ca
Departmental Affiliation(s): Biochemistry, Chemical Engineering
and Applied Chemistry, Institute of Biomaterials and Biomedical
Engineering
|
|
|
Rongmin
Zhao
Organisms such
as plants do not have the ability to move and escape from environmental
stresses and are more frequently affected adversely. Naturally,
plants like most other organisms develop complex mechanisms to sensor
and cope with environmental stresses in which processes the normal
protein folding and function are challenged. Our interests are to
understand how molecular chaperones, most of which are highly induced
to help the other proteins to function properly under stress conditions,
are involved in plant stress resistance and in the process of normal
plant development. Currently, the research is focused on the function
and the mechanism of action of molecular chaperone Hsp90 family
in the model plant Arabidopsis thaliana. On the other hand, we are
also interested in the role of selective protein degradation by
the proteasome in the control of cellular protein homeostasis. By
using yeast Saccharomyces cerevisiae as a model organism, we are
interested in studying how molecular chaperones are involved in
de novo protein synthesis and the interplay of chaperones and the
proteasome in the cellular protein quality control systems upon
protein misfolding by means of biochemical, biophysical and proteomics
approaches.
Website:
http://www.csb.utoronto.ca/faculty/zhao-rongmin
Departmental Affiliation(s): Biological Sciences, Cell and Systems
Biology
|
|